High Performance Fibers from Nature
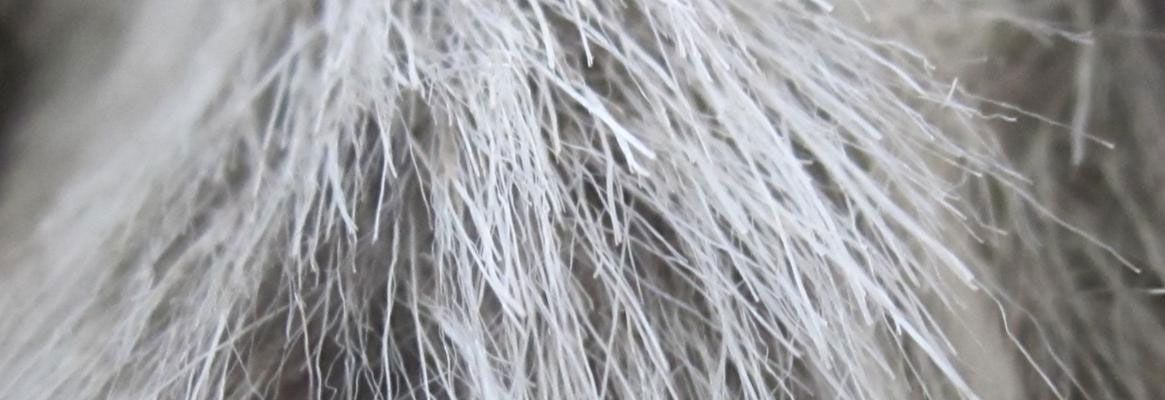
High Performance Fibers from Nature: Silk Processing and Assembly by Insects and Spiders'
Background
Silks are externally spun protein fibers generated by spiders and insects (Kaplan et aI., 1994). Reeled silkworm silk (Bombyx mori) has been used in the textile industry for over 5,000 years. Unlike silkworm silks, spider silk production has not been domesticated because spiders are more difficult to raise in large numbers due to their solitary and predatory nature. In addition, orb webs are not reelable as a single fiber and they generate only small quantities of silk. Silkworms can be raised in large numbers and generate one type of silk at one stage in their lifecycle, forming the basis for the sericulture industry. Many spiders have evolved families of silk proteins (different polymer chain chemistries- primary amino acid sequences) with different functions. For example, the spider, Nephila clavipes, generates at least six different silks from sets of different glands, each silk specifically matched to function- such as for environmental glues, strong or flexible web components, prey capture, and encapsulation (cocoons) for offspring development.
Silks are of interest for their remarkable mechanical properties as well as their durability, luster, and "feel." Silk fibers generated by spiders and silkworms represent the strongest natural fibers known, even rivaling synthetic high performance fibers in terms of mechanical properties (Gosline et al., 1986) The best properties of N. clavipes native dragline fibers collected and tested at quasi static rates were 60 and 2.9 GPa for initial modulus and ultimate tensile strength, respectively. In addition, these fibers display resistance to mechanical compression that distinguishes them from other high performance fibers (Cunniff et aI., 1994). Based on microscopic evaluations of knotted single fibers, no evidence of kink-band failure on the compressive side of a knot curve was observed. Synthetic high performance fibers fail by this mode even at relatively low stress levels. Silks are mechanically stable up to almost 200C (Cunniff et aI., 1994).
Spider dragline and silkworm cocoon silks are considered semi crystalline materials with the crystalline components termed b-sheets (Gosline et aI., 1986). Most silks assume a range of different secondary structures during processing from water-soluble protein in the glands to water-insoluble spun fibers. Marsh et al. (1955) first described the crystalline structure of silk as an anti-parallel hydrogen bonded b-sheet. The unit cell parameters in the silk II structure (the spun form of silk that is insoluble in water) are: 0.94 nm (inter chain), 0.697 nm (fiber axis), 0.92 nm (inter sheet). These unit cell dimensions are consistent with a crystalline structure in which the protein chains run anti parallel with inter chain hydrogen bonds perpendicular to the chain axis between carbonyl and amine groups, and van der Waal forces stabilizing the inter sheet interactions (based on the predominance of short side chain amino acids such as glycine, alanine, and serine in b-sheet regions). Generally, silkworm fibroin in cocoons contains a higher content of crystallinity (b-sheet content) than spider dragline silks such as from N. clavipes.
As more protein sequence data from various spiders and silkworms has been elucidated, it is clear that these families of silk proteins are similar but also encompass a range of sequence variations that reflect their functional properties. Silkworm fibroin is the protein that forms the structural aspects of the fibers. These fibers are encased in a family of glue-like sericin proteins. The primary sequence of amino acids of these proteins is found to be highly repeated, thus, small regions of sequence chemistry in the protein chain are found elsewhere in other regions of the chains. This design feature is critical to the function of this group of proteins as structural materials. This design feature also allows the sequences of these large proteins to be represented in relatively short sequences in terms of polymer design. This has led to the option of forming synthetic genetic variants to represent the larger proteins, a useful laboratory technique to enhance the ability to understand these proteins in a simplified form. These shorter genetic pieces can be polymerized (multimerized) into longer genes to explore sequence and size relationships.
These powerful tools in molecular biology facilitate direct insight into the role of sequence chemistry, protein block sizes and distributions, and protein polymer chain length on materials structure and function. Native and synthetic silk clones have been generated in a variety of heterologous expression systems, including bacteria, yeast, insect cells, plants, and mammalian cells (Wong and Kaplan, 2002).
Mechanism
Silk proteins are hydrophobic based on the predominance of glycine and alanine amino acids. The chains self-assemble into insoluble b-sheets. Spiders and silkworm keep these hydrophobic proteins soluble in water during their processing into fibers at concentrations up to 30 weight percent. The formation of liquid crystalline phases during silk processing has been reported as part of this process (Vollrath and Knight, 2001). This process is accomplished in vivo without premature crystallization into the insoluble b-sheets. Premature crystallization would be catastrophic for the animal as it would clog the spinning device. In recent studies, micelle and gel states were identified that suggest these are important steps governing chain silk protein interactions toward organized silk structures.
These features can be duplicated in part using regenerated silkworm silk and the control of water removal from aqueous solutions of these proteins via osmotic stress (Jin and Kaplan 2003). These "soft" micelles consist of flexible molecules and the structures can grow and change shape in response to changes in protein concentration. This is typical behavior of amphiphilic flexible surfactants that assemble into small spherical micelles and evolve morphologically into cylinders and lamellar-layered structures. Lyotropic liquid crystalline phase behavior can help explain the assembly of silk protein polymer chains into domains of high concentration (Vollrath and Knight, 2001), with orientation driven by micellar behavior and water efflux through channels in these structures.
Based on the micellar behavior and subsequent morphological features generated during silk processing, critical design rules (chemistry of the amino acid sequence and blocks or regions of the sequence chemistry) to match the processing environment have been described (Bini et aI. 2004). These design rules demonstrate a modified tri-block design for these proteins that also contrasts in important ways with traditional synthetic tri-block co-polymers. In silks, the large (dominating in size and chemical influence) internal hydrophobic blocks (crystallizable domains which promote intra and interchain folding) are interrupted with very short hydrophilic blocks or spacers, putatively to control water content in micellar states to prevent premature crystallization into b-sheets. The large N-and C-terminal hydrophilic blocks interact with water and define micellar partitioning. All protein sequences in silks adopt these general block "design rules" (Bini et aI, 2004) presumably to match the limits of the all aqueous processing environment.
There is, however, significant sequence (chemistry) variability permitted in the hydrophobic or dominant regions of the protein which give rise to the variations in functional properties of the different silks. In the final step of the process of formation of silk fibers, the assembled proteins are spun through orifices in the abdomen of spiders or mouth of silkworms, inducing the structural and morphological transition of the gel state from the gland into the b-sheet structure and fiber morphology. The spun fibers are Insoluble in water.
In general, the combined control of sequence chemistry and processing conditions is central to the successful formation of high-performance fibers based on silk proteins. Included in this is the presence of suitable blocks and chemical features of these blocks to deal with the processing environment and the appropriate mechanical properties. To date, no successful example has been reported of spinning recombinant or reconstituted silk proteins that emulate the full range of novel mechanical properties of silkworm or spider silk fibers.
Mimetic Systems
Many aspects of the silk spinning process can be mimicked in vitro and the all-aqueous environment used is instructive as a model for polymer processing, in general. Employing new insights into silk fibroin solubility and assembly has permitted new forms of these protein-based materials to be generated. For example, porous 3-D sponges have been formed from regenerated silk fibroin (Nazarov et al.. 2004). Blending silk fibroin and polyethylene oxide to obtain sufficient solution viscosity suitable for electro-spinning to generate nanoscale-diameter fibers has also been reported (Jin et aI., 2002). The insights into silk protein assembly have been utilized toward the formation of patterned peptide multilayer thin films with nanoscale order (Valluzzi et aI., 2003).
This engineered liquid crystallinity to form smectic layers was generated with thin films and bulk materials, with tunability of the layer thickness and patterning based on sequence design and chain length. In addition to recapitulation of the silk assembly process in vitro as outlined above based on new insight into the process of silk protein-protein interactions, efforts are underway in many laboratories to develop synthetic analogs of silk. For example, copolymers of glycine-alanine repeats (hard segments, b-sheet formation) with polyethylene glycol (soft segments) have been studied to provide fundamental insight into control of polymer assembly and structure (Rathore and Sogah, 2001).
With continuing advances in genetic engineering, improved quantities and the availability of additional sequence variants of silk proteins can be anticipated. These materials, combined with new understanding of aqueous processing of these polymers, should help with continued improvements in the ability to generate silk-based materials for a wide range of potential utility. At the same time, novel polymer mimics of these systems in which the key design rules are considered will continue to emerge. Finally with the wealth of silk protein sequence-structure-function data being generated from the study of different silks, this family of unusual protein polymers can serve as a blueprint for future designs and engineering to develop synthetic analogs using more traditional synthetic polymer approaches.
Spider Web -Strong Fibers:
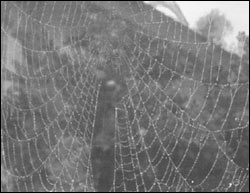
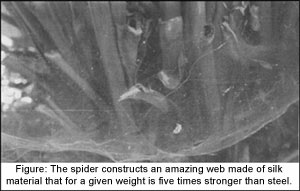
The spider generates the fiber while at the same time hanging on to it as it emerges cured and flawless from its body. The web is generated at room temperature and at atmospheric pressure. The spider has sufficient supply of raw materials for its silk to span great distances. It is common to see webs spun in various shapes (including flat) between distant trees, and the web is amazingly large compared to the size of the spider. Another interesting aspect of the spider web is the fact that it is a sticky material intended to catch prey, but the spider itself is able to move freely on it without being trapped.
The silk that is produced by a spider is far superior in toughness and elasticity to Kevlar, which is widely used as one of the leading materials in bullet proof vests, aerospace structures, and other applications where there is a need for strong lightweight fibers. Though produced in water, at room temperature and pressure, spider's silk is much stronger than steel. The tensile strength of the radial threads of spider silk is 1154 MPa, while steel is 400 MPa (Vogel, 2003). Spiders eat flies and digest them to produce the silk that comes out from their back ends, and spool the silk as it is produced while preparing a web for trapping insects.
This web is designed to catch insects that cross the net and get stuck due to its stickiness and complexity. While the net is effective in catching insects, the spider is able to maneuver on it without the risk of being caught in its own trap. Recent progress in nano-technology reveals a promise for making fibers that are fine, continuous, and enormously strong. For this purpose, an electro spinning technique was developed (Dzenis, 2004) that allows producing 2-mm diameter fibers from polymer solutions and melts in high electric fields. The resulting nano-fibers were found to be relatively uniform without requiring extensive purification.